Cardiac fibrillation describes the deterioration of the heart’s electrical activity, which drives the heart’s periodic pumping. This electrical activity, which is usually organized, becomes disorganized into self-sustained patterns of electrical activation.
Cardiac fibrillation leads to a reduction in cardiac output that can quickly cause death in the absence of clinical intervention. Cardiac defibrillation using an implantable and automatic device to deliver a strong electric shock to the heart is the only effective technique for treating life threatening disturbances in cardiac rhythm.
Accurate 3D models of ventricular defibrillation, along with close experimental observation have been key to uncovering the electrical events that occur as a result of the interaction between fibrillating myocardium and the administration of electrical shock.
The effectiveness of a cardiac defibrillator very much depends on the positioning of the electrodes that transmit electrical currents to the heart. Most implanted defibrillators are used to treat people aged 80 years or over, but sometimes they need to be used to treat children. The positioning of a defibrillator is particularly important where children are concerned because their growth may gradually change the positioning. The anatomy of the child’s heart also differs from that of the adult heart.
Software modelling systems now exist to determine the optimal positioning of defibrillators. Modelling systems can be used to map the thorax and indicate the prime positioning of either internal or external cardiac defibrillators, thereby increasing their efficacy.
This software uses surgical planning applications along with myocardial voltage gradients to predict the likelihood of the defibrillator being successful. If the critical mass hypothesis is applied, effective defibrillation is only achieved if a threshold voltage gradient is produced in a large proportion of the heart muscles. Specifically, a gradient of 3 to 5 volts per centimetre in 95% of the heart is usually necessary. Gradients over 60 volts per centimetre can damage heart tissue and the software is designed to obtain safe gradient values that are still above the threshold for successful defibrillation.
Simulation studies using this software have shown that even small changes in the positioning of electrodes may have significant effects on defibrillation. The modelling software systems provide guidance in choosing the optimal placement of defibrillators in children and adults.
Bidomain modelling
Recent designs are based on bidomain models of cardiac tissue, to provide a realistic heart shape and geometry of heart fibres for determining how cardiac tissue responds to electric shock.
In one study using the bidomain model of the myocardium, which accounts for the flow of electrical current in both interstitial and intracellular domains, Sepulveda et al., showed membrane polarization induced by shock may be more complex than was previously thought.
Their simulation study indicated that the myocardium tissue response to a strong uniploar stimulus involved depolarizing and hyperpolarizing effects that occur in close proximity if both the intracellular and extracellular spaces are anisotropic (directionally dependent), but to a different degree. In monodomain models, equal anisotropies are inherently assumed and it is not possible for polarizations of opposite polarity to occur. The possibility of these “virtual electrodes” existing was later followed up using optical mapping, which confirmed the prediction. “Virtual electrode polarization” (VEP) has since become a mainstay in defibrillation research. VEP in the myocardium now refers to the areas of electric shock-induced membrane depolarization and hyperpolarization and their spatial pattern in the ventricles, which represents the new myocardial state at the end of the shock. The post-shock electrical activity that follows is largely dependent on this new state of the myocardium.
Bidomain modelling studies have shown that the post-shock VEP pattern is the main factor determining the origin of post-electric shock activations in the second stage of the defibrillation process. In regions where electric-shock induced virtual anodes and cathodes are in close proximity, it is possible to elicit a “break” excitation after the shock has ended. Depolarized cells in the cathode provide an excitatory current that induces a regenerative depolarization and propagating wave in the newly created virtual anodes within immediate proximity. Positive VEP can also result in straightforward depolarization of the excitable gaps present when the shock is delivered to the fibrillating myocardium. These are referred to as “make” excitations and their discovery, along with that of break excitations has led to an improved understanding of how new activations develop as the result of administering a strong stimulus.
Sources
- www.cchaforlife.org/…/ACHD-Pacemakers-and-Defibrillators.pdf
- http://www.who.int/medical_devices/innovation/defibrillator_manual.pdf
- www.heart.org/…/ucm_300340.pdf
- https://www.resus.org.uk/pages/aed.pdf
- http://europace.oxfordjournals.org/content/16/5/705
Further Reading
- All Defibrillator Content
- Defibrillator Types
- Defibrillator – What is a Defibrillator?
- How to use a Defibrillator
Last Updated: Aug 23, 2018
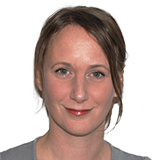
Written by
Sally Robertson
Sally has a Bachelor's Degree in Biomedical Sciences (B.Sc.). She is a specialist in reviewing and summarising the latest findings across all areas of medicine covered in major, high-impact, world-leading international medical journals, international press conferences and bulletins from governmental agencies and regulatory bodies. At News-Medical, Sally generates daily news features, life science articles and interview coverage.
Source: Read Full Article